Newly Discovered Transient Pressure Surge Effect May Be Underlying Mechanism for Earthquake Dynamic Triggering
Earthquakes are natural phenomenon posing significant hazards to society. Following a major earthquake, it is common for aftershocks to occur within tens of kilometers of the main shock for weeks or years.
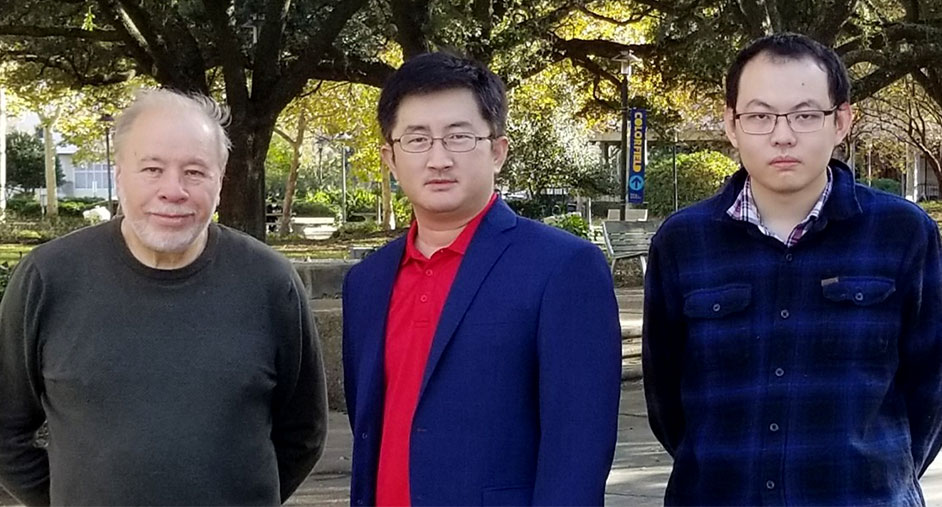
However, in a lesser known phenomenon, called earthquake dynamic triggering, the seismic waves from the main shock can trigger earthquakes in regions containing fluids, such as geothermal and volcanic fields, thousands of kilometers away from the main shock. Although less familiar to the public, earthquake dynamic triggering is a well-established observation in seismology.
“These seismic-wave triggered earthquakes are different than induced seismicity, where earthquakes are caused because of injection of water or other fluid into the rock,” said Yingcai Zheng, the Robert and Margaret Sheriff Professor in Applied Geophysics at the University of Houston’s Department of Earth & Atmospheric Sciences.
In dynamic triggering, no substance is injected into the ground; the earthquakes are triggered by the stress perturbation carried by the passing seismic waves. Several hundred triggered cases have been documented so far.
When calculated, the stress carried by the seismic waves after propagating thousands of kilometers is quite low. In scientific terms, it’s a few thousand Pascal or 1% of the atmospheric pressure. That’s like the pressure caused by a stack of 10 U.S. quarters on your hand.
“How such a small stress perturbation can cause earthquakes has been a puzzle in seismology,” Zheng said.
In a paper published in The Seismic Record, UH researchers describe a new triggering mechanism observed in a laboratory experiment, which answers the puzzling question of earthquake triggering. The paper, “Laboratory Evidence of Transient Pressure Surge in a Fluid-Filled Fracture as a Potential Driver of Remote Dynamic Earthquake Triggering,” is authored by Yuesu Jin (first author), a UH graduate student, and Nikolay Dyaur, a retired UH research scientist, who is now at the Russian Academy of Sciences, and Zheng.
Traditional wisdom says the earth’s crust is in a critically stressed state and is ready to fail, causing earthquakes any time. In this sense, the seismic wave is the last straw that broke the camel’s back. However, Zheng says there are some issues about this critical state interpretation.
“First, low-frequency waves, usually the surface waves, have more potential to trigger earthquakes than high-frequency body waves. Secondly, the triggered earthquakes are preferentially located in regions having fluids such as in geothermal and volcanic areas,” he said.
In 2018, Zheng published a paper on numerical modeling seismic wave interaction with a fluid-filled fracture. He found that the fluid pressure in the fracture could be amplified by more than a few hundred times relative to the incoming seismic wave pressure.
“For a finite-sized thin fluid-filled fracture, the two fluid-solid boundaries can exhibit interfacial phenomenon and can significantly slow down the waves. This slow down results in large pressure build up and amplification in the fracture. It’s like fast traveling cars a highway suddenly need to slow down to result in a traffic jam,” Zheng said.
The amplification factor depends on both the wave frequency and the fracture geometry and aperture. Zheng called this the “transient pressure surge effect.” Such a significant pressure amplification can reduce the confining stress acting on the fault surfaces, causing them to slip and resulting in triggered earthquakes.
With National Science Foundation funding, Zheng set out to verify the pressure surge effect in the lab using physical experiments in 2018.
“I began to realize that I underestimated the challenges in doing the experiment,” Zheng said. “After we worked on the experiment for a while, we realized there were no seismic sources and receivers on the market that fit our needs.”
“According to our numerical calculation, the pressure surge effect can be observed in the low-frequency regime using bigger blocks, so we began to design and build a low-frequency underwater experiment platform from scratch, including our low-frequency source and our new pressure sensors that can be placed in a thin-fracture.” said Jin, who is part of Zheng’s research team.
The meter-scale fracture model was made by stacking two large plexiglass blocks. Electronic design and circuits, mechanical design and manufacture, and control software are all part of the low-frequency experimental system.
The low-frequency source (Xfrac-S) can excite sinusoidal acoustic waves in water, and the pressure sensors placed both outside and inside the fracture recorded the dynamic pressure signals. The Xfrac-H pressure sensor is a flexural type sensor ~0.2 millimeters thick (like an eardrum) which can be placed in thin fractures to measure fluid pressure.
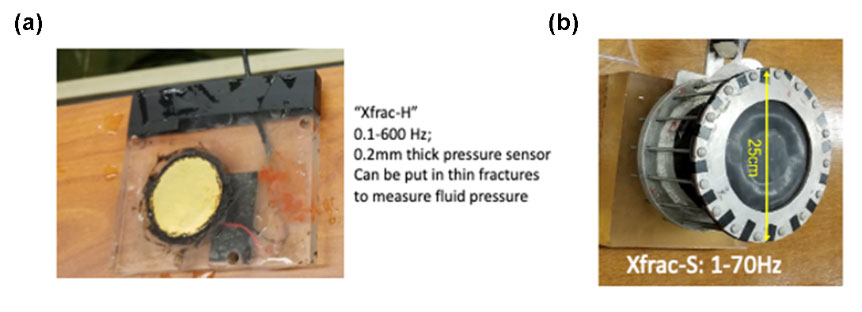
“During the COVID pandemic, we focused on building the whole low-frequency experiment system and calibrating the low-frequency source and transducers. We finished the calibration process and observed the pressure surge again and again,” Jin said.
This low-frequency system is unique, and it allowed the researchers to directly observe the physics inside the thin fractures.
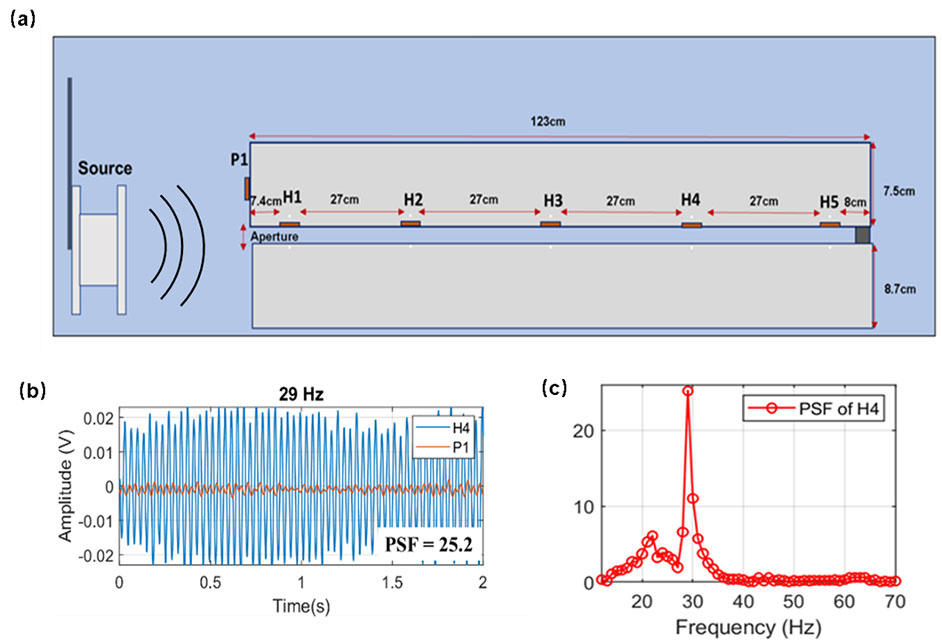
To measure the amount of amplification, the team defined the pressure surge factor (PSF) as the ratio between the pressure inside and outside the fluid-filled fracture. The UH team got the maximum PSF as 25.2 using the meter scale glass model with a 0.4mm fracture aperture, when the seismic wave frequency is 29Hz.
“This observation shows seismic waves can indeed amplify fluid pressure in fractures. It was an important observation,” Zheng said.
“For example, if a stress carried by a seismic wave is 0.1MPa, after the interaction with the fracture, the fluid pressure in the fracture can achieve up to 2.5MPa, enough to trigger earthquakes,” Jin said.
In reality, the fracture can be longer, the pressure surge factor might be more than 100, which enables a tiny stress perturbation to trigger the earthquake in fluid and fracture many regions.
“Our research is not done yet. The current fracture model uses glass blocks, not real rock. The dimension of the fracture is only 1m scale. We need a bigger fracture model and realistic rough fracture surfaces or multiple fractures of different scales to see if we can still see the pressure surge effect,” Zheng said.
Future field testing of the pressure surge mechanism is necessary for its wide applications. If the pressure surge effect can be verified in the field, this fundamental research can open a set of new research directions.
For example, it can be used to image and characterize the location, size, and geometry of the subsurface fractures. The localized pressure increase can also be used to alter fluid flow in the subsurface. Both are important in oil/gas and geothermal energy.
One can also use pressure surge effect to probe the earthquake failure stress threshold because different wave frequencies cause different amounts of stress changes on the fault. Such information is critical in predicting earthquake hazards and mitigation.